Introduction
Photonic integrated circuits (PICs) have emerged as a pioneering technology, enabling high-speed data transmission, quantum computing, and artificial intelligence acceleration. At the heart of these advancements lie programmable building blocks that can dynamically reconfigure the behavior of the PIC. Researchers have explored various approaches, including Mach-Zehnder Interferometers (MZIs), micro-ring resonators, and directional couplers. In a recent development, a multi-mode waveguide-based beam splitter (MMWBS) has been proposed and demonstrated, offering an innovative solution for programmable photonic circuits.
Working Principle
The MMWBS is composed of two key elements: a multimode waveguide (MMW) and two compact mode converters. The mode converter, as illustrated in Fig. 1(a), is designed to split the MMW into two single-mode waveguides (SMWs) through an inverse-designed region.
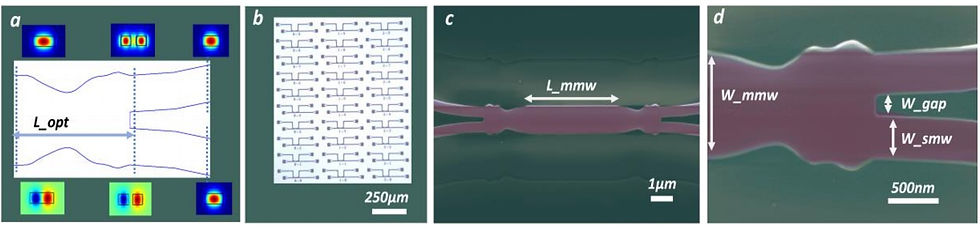
This mode converter acts as a 50:50 coupler between the two SMWs and the TE0 and TE1 modes of the MMW. The system, as shown in Fig. 1(c), is analogous to a Mach-Zehnder interferometer, with two optical paths formed by the TE0 and TE1 modes. The splitting ratio at the output end of the MMW is determined by the optical path difference between these two modes, which is a function of the MMW length (L_mmw).
Device Design and Fabrication
The MMWBS was fabricated on a silicon-on-insulator (SOI) wafer using electron-beam lithography and inductively coupled plasma (ICP) etching. Fig. 1(b) shows a micrograph image of the fabricated devices, while Fig. 1(c) and 1(d) provide scanning electron microscope (SEM) images of the MMWBS and mode converter, respectively.
Simulation and Experimental Results
Simulations revealed promising results for the MMWBS. The insertion loss (IL) of the mode converter was lower than (-0.15, -0.18) dB for (TE0, TE1) modes, as shown in Fig. 2(a). The splitting ratio of the MMWBS varies sinusoidally with the length of the MMW, as depicted in Fig. 2(b), allowing for the realization of arbitrary split ratios.
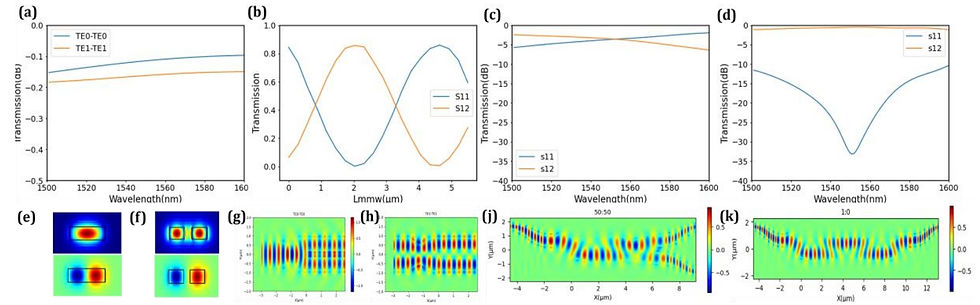
The transmission spectra for the MMWBS designed with 50:50 and 1:0 splitting ratios are illustrated in Fig. 2(c) and 2(d), respectively. Fig. 2(e) shows the TE0 and TE1 modes of the MMW, while Fig. 2(f) displays the two super modes when two SMWs are closely packed together. The propagation electric fields for the mode converter and the MMWBS with 50:50 and 1:0 splitting ratios are presented in Fig. 2(g)-(h) and Fig. 2(j)-(k), respectively.
Experimental validation of the MMWBS yielded remarkable results. Fig. 3(a) shows the measured and fitted splitting ratio of the MMWBS as a function of the MMW length, exhibiting excellent agreement with the simulations in Fig. 2(b).
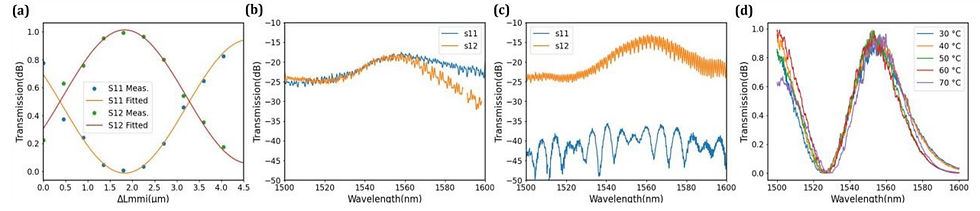
The transmission spectra for the MMWBS with 50:50 and 1:0 splitting ratios are depicted in Fig. 3(b) and 3(c), respectively. The measured IL of the MMWBS was lower than -0.5 dB, and the extinction ratio for the 1:0 switch exceeded 25 dB, demonstrating remarkable performance. Furthermore, the device exhibited temperature insensitivity, as shown in Fig. 3(d), where the transmission spectrum remained unchanged when the temperature of the PIC was varied from 30°C to 70°C.
Applications and Future Prospects
The MMWBS presents exciting opportunities for programmable photonic integrated circuits. By combining thermally sensitive and insensitive MMWBSs, tunable beam splitters and phase shifters can be realized, respectively. These elements can be integrated as programmable unit cells, providing equivalent functionality to MZIs and enabling a wide range of applications in areas such as deep learning optical accelerators, integrated quantum photonics, and optical communication systems.
Future research could explore the integration of MMWBSs with other photonic components, such as modulators and detectors, to create fully programmable PICs. Additionally, the scalability of MMWBS-based circuits and their potential for large-scale integration and miniaturization could be investigated.
Conclusion
The multi-mode waveguide-based beam splitter (MMWBS) represents a significant advancement in the field of reconfigurable photonic circuits. By harnessing the interference between TE0 and TE1 modes in a multimode waveguide, arbitrary splitting ratios can be achieved with low insertion loss and high extinction ratios. The temperature-insensitive MMWBS demonstrated in this work paves the way for programmable unit cells that can be integrated into larger photonic circuits, enabling a wide range of applications in areas such as optical computing, quantum information processing, and telecommunication systems.
Reference
[1] D. Yi and H. K. Tsang, "Towards Reconfigurable Photonic Circuits Based on Multimode Waveguides," Department of Electronic Engineering, The Chinese University of Hong Kong, Shatin, NT, Hong Kong SAR, China, 2023, pp. 1-6, doi: 979-8-3503-9404-7/24/$31.00 ©2023 IEEE.
Commentaires