OFC2025|Diagnosis of Aerial Telecom Facilities Using High-Precision Φ-OTDR DAS Technology
- Latitude Design Systems
- 12 minutes ago
- 4 min read
Introduction
Maintenance and monitoring of telecommunications infrastructure are crucial to ensuring reliable service. Traditional monitoring technologies rely on optical time domain reflectometry (OTDR) to detect anomalies in telecom optical fibers. However, recent advancements in phase-sensitive OTDR (Φ-OTDR)–based distributed acoustic sensing (DAS) offer new methods for intelligent maintenance and operation of optical communication facilities [1].
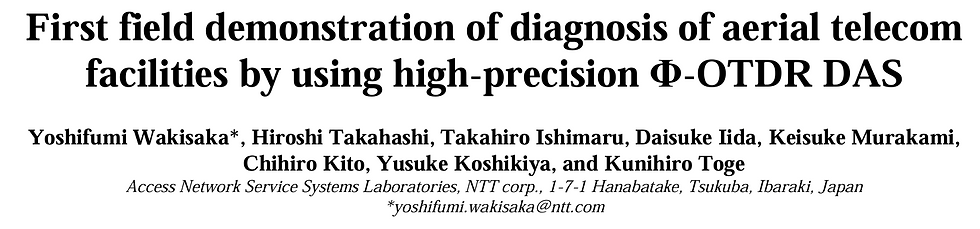
Overview of Optical Fiber Monitoring Technologies
OTDR has long been a fundamental tool for detecting anomalies in telecom optical fibers that may lead to communication failures. Conventional OTDR can detect events such as bending and reflection that cause signal loss and can even serve as an early warning system for water ingress in manholes. With the advent of phase-sensitive OTDR (Φ-OTDR), which enables long-distance DAS, the range of potential applications has significantly expanded. Various use cases have been demonstrated, particularly for underground infrastructure, including manhole site identification, cable routing detection, and threat identification.
However, Φ-OTDR DAS faces limitations, such as location-dependent strain sensitivity due to fading and moderate spatial resolution (on the meter scale) defined by the detection pulse width. These limitations make extracting meaningful information from field trials challenging. While solutions using advanced data analysis with AI and ML have been proposed, Φ-OTDR applications remain underexplored.
Challenges on Monitoring Aerial Cables
Aerial cables face unique challenges as they are continuously exposed to harsh weather conditions such as wind and rain. Irreversible anomalies such as sagging and detachment from bundled hangers may occur. If left unaddressed, these issues can disrupt communication services and potentially cause severe accidents involving people, vehicles, and buildings. Early detection is essential, but visual inspection across extensive aerial networks demands significant manpower and time.

Unlike sagging that occurs between utility poles, hanger detachment happens in specific sections within the span. This makes early-stage detection difficult, particularly if fading noise is still present, as the affected section’s strain sensitivity may be degraded.
Multi-Frequency Φ-OTDR Technology
To address the challenge of fading noise, researchers adopted multi-frequency Φ-OTDR. By averaging multiple frequency signals, they effectively suppressed fading. The study employed a vector-based phase averaging method capable of computing large vibration waveforms exceeding sub-microstrain with high precision and accuracy, suitable for measuring wind-induced swaying of aerial cables.
The experimental setup used a single-sideband modulator (SSBM) to multiplex 8 frequencies. Each pulse width was 40 ns, providing a spatial resolution of 4 meters. The optical signal-to-noise ratio (OSNR) was enhanced using amplifiers (SOAs, EDFAs). The backscattered signals received by the coherent receiver were processed with digital band-pass filters to compute multi-frequency signals on the IQ plane.
In vector-based averaging, differences in phase offset are tracked and compensated using a time window that matches the vibration measurement timescale. For this study, researchers set the window to 10 ms (100 Hz), sufficient to observe critical vibration signals while maintaining strain sensitivity.
Field Demonstration and Experimental Setup
Researchers used a 4.3 km-long field-deployed telecom service fiber cable. The aerial section started at approximately the 2 km point, with hanger detachment occurring within a 40 m span around the 2.5 km mark. Measurements were conducted before and after repair at multiple time points (days and time periods), including immediately after repair and 10 months later.
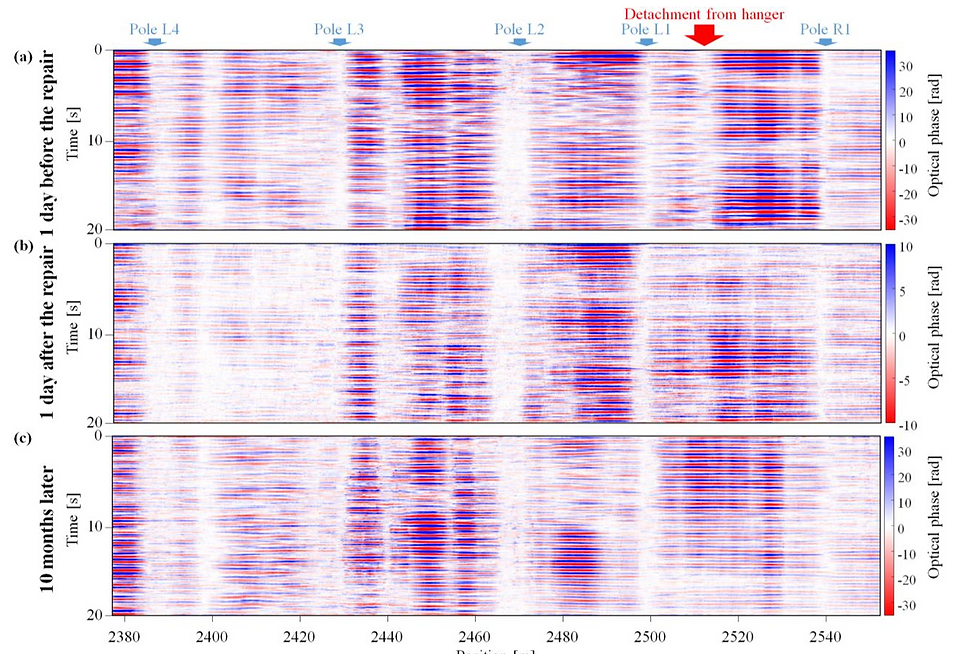
Results and Analysis
Comparative waterfall plots before and after repair reveal a magnified view of spatiotemporal patterns. While wind conditions varied daily (as evidenced by differences in vibration amplitude), similar patterns were observed. Researchers first examined utility pole locations and confirmed that vibration propagation patterns changed at pole positions—showing lower vibration amplitude and significant phase variation along the spatial axis.
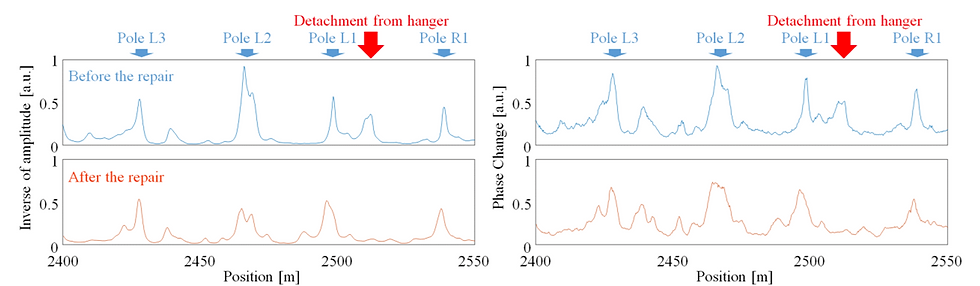
By calculating and averaging the amplitude and phase variation of vibrations along the fiber (normalized every 20 seconds), researchers found a strong correlation between the two parameters. This indicated that utility pole positions could be identified using these parameters—marking the first demonstration of pole identification using Φ-OTDR.
Notably, the same parameters proved useful for detecting hanger detachment. As shown in Figures 2 and 3, before the repair, the vibration pattern of the detached segment changed similarly to that at the pole location. After the repair, this trend became less apparent.
Frequency Analysis for Enhanced Detection
To investigate further, frequency analysis was performed. Averaged spectra from multiple data points within the same span—before, at, and after detachment—revealed that while absolute intensities varied, the strongest natural vibration frequency (NVF) peak at 1.3 Hz and the secondary peak at 2.1 Hz appeared across all spectra.
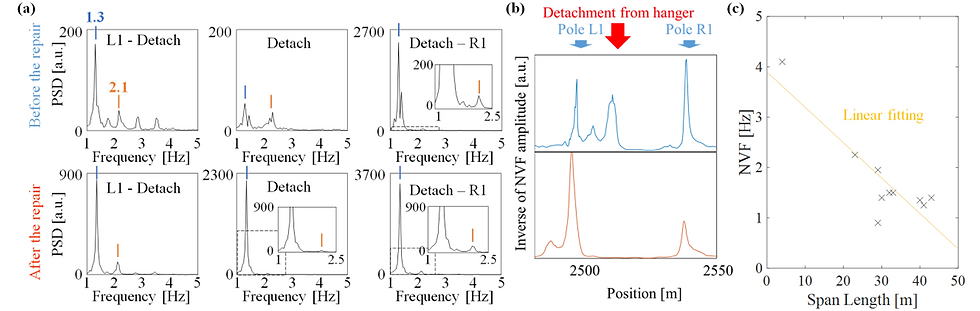
In the detached segment before repair, the second peak did not significantly decrease, but the NVF peak was relatively small and comparable to the second peak, creating a unique spectral signature. Based on this observation, monitoring NVF amplitude variations improved signal visibility—more effectively than simple amplitude analysis.
The correlation between span length and NVF showed similar NVF signals (typically 1–2 Hz) in other spans as well, indicating broader applicability. Importantly, a negative correlation was observed, highlighting the need to assess and apply appropriate NVF values in practical operations across various span lengths.
Conclusion
The first field demonstration of diagnosing aerial cables using multi-frequency Φ-OTDR DAS yielded promising results. By effectively suppressing fading, researchers demonstrated that vibration pattern parameters can identify utility pole locations even at moderate resolution. These same parameters were useful in detecting hanger detachment at an early stage, while NVF analysis enhanced signal visibility.
These findings indicate that long-distance Φ-OTDR DAS has significant potential for diagnosing aerial telecom facilities and early anomaly detection, potentially revolutionizing maintenance practices for telecom infrastructure.
Reference
[1] Y. Wakisaka, H. Takahashi, T. Ishimaru, D. Iida, K. Murakami, C. Kito, Y. Koshikiya, and K. Toge, "First field demonstration of diagnosis of aerial telecom facilities by using high-precision Φ-OTDR DAS," in Optical Fiber Communication Conference (OFC) 2025, paper M1C.4, 2025.
Comments